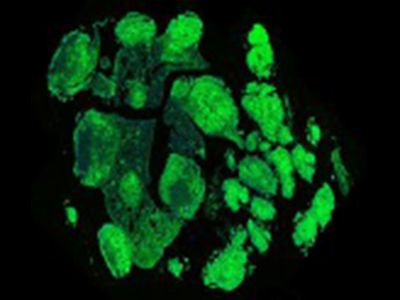
Many tumours are successfully treated with surgery. But cancer might recur at the surgical site or in distant organs, so surgery is often followed by treatment termed adjuvant therapy. This limits the risk of relapse by killing cancer cells remaining at the surgical site or those that have already moved elsewhere. However, adjuvant therapy is not always effective. Moreover, it might not prevent certain processes that aid cancer resurgence, such as the recruitment of blood cells called myeloid cells to distant organs, where they can lay the foundation for cancer cells to settle and thrive1. Writing in Nature, Lu et al.2 reveal how the chemical structure of the DNA in the nucleus of myeloid cells is a vulnerability that can be harnessed to target these tumour-promoting cells and limit cancer spread.
Tumour spread from its primary site to distant organs, which is called metastasis, involves complex interactions between cancer cells and the surrounding healthy tissues. Evidence is growing that primary tumours can produce signals that modify normal cells to generate a ‘soil’ in distant organs — termed a pre-metastatic niche — that permits subsequent ‘seeding’ and establishment of cancer cells at this secondary site3. Such secondary tumours, or metastases, are often lethal.
Several types of cancer metastasize from their primary site to the lung. Efficient metastasis of breast cancer cells to the lung in mice requires the participation of a type of myeloid cell called a monocyte1,4. These cells normally function to fight infections, but they can also supply metastasizing cancer cells with factors that help them to get established and grow at a secondary site1,4. Certain molecules produced by the primary tumour alter the properties of monocytes and increase their numbers in the bloodstream, fostering the monocytes’ tumour-supporting functions in the pre-metastatic niche1,4. Therefore, blocking such ‘tumour-educated’ monocytes might inhibit metastasis. Previous work1,4 indicates that neutralizing a protein called CCL2, which is produced by the tumour and promotes monocyte accumulation in the lungs of mice, impairs metastasis in mouse tumour models. However, this approach was unsuccessful in clinical trials owing to difficulties in effectively neutralizing CCL24.
Lu et al. studied mice that were given transplants under the skin of a type of tumour that metastasizes to the lung. Consistent with previous studies4,5, the authors found that two types of myeloid cell — monocytes and neutrophils — accumulated in the lung before metastases were detectable there. These tumour-elicited myeloid cells are collectively called myeloid-derived suppressor cells (MDSCs) owing to their ability to suppress the immune response against a tumour5. Confirming the metastasis-promoting capacity of MDSCs, the authors report that elimination of MDSCs delayed the metastasis of cancer cells to the lung and extended the animals’ survival.
Gene expression can be regulated by changes in the nucleus termed epigenetic modifications. These include processes that add or remove either methyl groups on DNA or acetyl groups on DNA-binding proteins called histones. Such epigenetic modifications often alter the expression of many genes in cancer cells, which might lead to loss of expression of genes that function as tumour suppressors, or to high expression of tumour-promoting genes. Anticancer treatments that use drugs to modulate epigenetic changes (termed epigenetic therapies) are gaining momentum6. Some of these drugs have been tested in clinical trials and approved for the treatment of certain blood tumours6.
Epigenetic therapy is usually aimed at cancer cells, but earlier work7,8 indicates that such treatment can also target tumour-promoting myeloid cells. Lu and colleagues investigated the effect of using low doses of two such drugs: 5-azacytidine (which inhibits the addition of methyl groups to DNA) and entinostat (which inhibits the removal of acetyl groups from histones). This treatment did not kill cancer cells in vitro, nor did it inhibit the growth of primary tumours in mice. However, when mice that had undergone tumour-removal surgery were treated with the drugs, the migration of myeloid cells to the lungs and the formation of lung tumours were reduced (Fig. 1) compared with the effects in mice that did not receive the drugs. Lu and colleagues report that a similar treatment is well tolerated by people who have lung cancer, and preliminary evidence presented by the authors also suggests that it might limit tumour recurrence after surgery.
Myeloid cells are made in the bone marrow and released into the bloodstream. Lu and colleagues report that epigenetic therapy modulated the gene expression of myeloid cells in the bone marrow of mice. By altering the chemical structure of DNA, epigenetic drugs can modify the ability of transcription-factor proteins to bind DNA and promote the expression of certain genes.
Epigenetic therapy suppressed the activity of a transcription factor called NF-κB in the marrow-resident myeloid cells. NF-κB controls the expression of CCR2 (which is the receptor for CCL2) and CXCR2 (which is the receptor for another tumour-derived protein called CXCL1). After treatment, monocytes expressed lower levels of CCR2, and neutrophils expressed lower levels of CXCR2. CCL2 and CXCL1 drive the recruitment and retention of myeloid cells in the lung4,5. Therefore, drug-induced inhibition of CCR2 and CXCR2 expression probably impaired the process that enables a pre-metastatic niche in the lung to be populated by tumour-promoting myeloid cells.
Lu et al. observed that the drug-mediated epigenetic reprogramming of myeloid cells was maintained during their exit from the bone marrow and when they reached the lung. Indeed, in addition to NF-κB, the activity of several transcription factors known to orchestrate the generation of a pre-metastatic niche3 was suppressed in the myeloid cells that made their way to the lung. Intriguingly, the authors found that the epigenetic therapy caused monocytes to differentiate into a type of cell called an interstitial macrophage, which might lack the ability to promote tumour establishment at a secondary site. The drugs also caused this type of cellular conversion in monocytes that were experimentally transferred from the bone marrow of untreated mice to the bloodstream of drug-treated mice. How the drugs drive this change is unknown. Perhaps their ability to rewire a cell’s transcriptional network underlies this phenomenon.
Lu and colleagues’ findings indicate that the re-education of tumour-hijacked myeloid cells using epigenetic therapy might be a promising strategy for thwarting their metastasis-promoting capacity. These cells have several tumour-promoting functions, such as boosting the formation of blood vessels that aid tumour growth or suppressing antitumoral T cells of the immune system5,9. Thus, rather than having to individually target factors that aid different processes needed for tumour growth, epigenetic therapy might be a way to neutralize the broad and multifaceted capacity of myeloid cells to aid cancer.
Drugs such as 5-azacytidine and entinostat are being tested in the clinic for use in combination with other anticancer therapies, both before tumour-removal surgery and in the setting of adjuvant therapy after surgery10. These drugs are not approved yet for treating solid (non-blood-cell) tumours in the clinic. High doses of such drugs are associated with severe toxicity, so using low doses that suffice to reprogram myeloid cells, together with other drugs that target cancer cells, might provide an effective anticancer treatment that avoids unwanted toxicity.