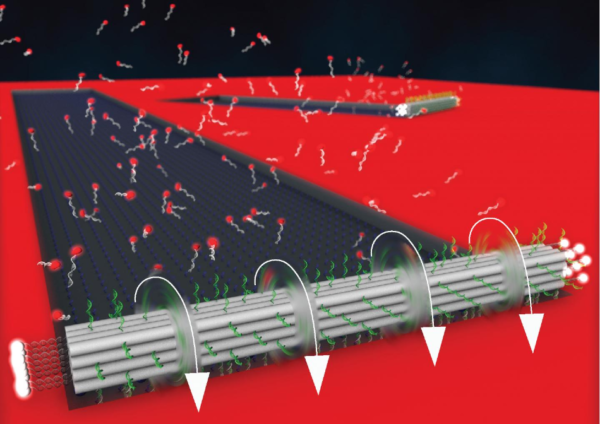
Written by AZoNanoMar 4 2020
Researchers have developed the world’s fastest and most persistent DNA nano motor using a technique called DNA origami.
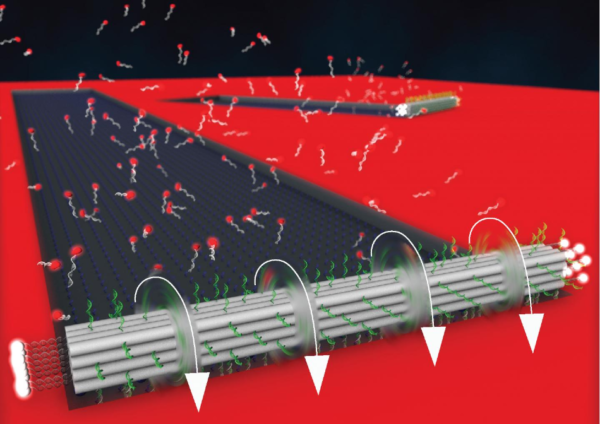
The findings, published in Angewandte Chemie, provide an outline on ways to improve the design of nano motors at the nanometer scale—that is, many times smaller than the normal human cell.
Nanoscale motors have tremendous potential for applications in biosensing, in building synthetic cells and also for molecular robotics. DNA origami allowed us to tinker with the structure of the motor and tease out the design parameters that control its properties.
Khalid Salaita, Study Senior Author and Professor, Department of Chemistry, Emory University
Using RNA fuel, the new, rod-shaped DNA motor rolls persistently in a straight line at a pace of up to 100 nm per minute and without any human intervention, that is, up to 10 times quicker than the speed of earlier DNA nano motors.
Salaita is on the faculty of the Wallace H. Coulter Department of Biomedical Engineering, which is a collaborative program of Emory University and Georgia Institute of Technology. The study is a joint effort between the Salaita laboratory and Yonggang Ke, assistant professor at Emory University’s School of Medicine and the Wallace H. Coulter Department of Biomedical Engineering.
Our engineered DNA motor is fast, but we still have a long way to go to achieve the versatility and efficiency of nature’s biological motors. Ultimately, the goal is to make artificial motors that match the sophistication and functionality of proteins that move cargo around in cells and allow them to perform various functions.
Yonggang Ke, Assistant Professor, School of Medicine and Wallace H. Coulter Department of Biomedical Engineering, Emory University
Generating things out of DNA, dubbed DNA origami after the conventional Japanese paper folding craft, leverages the natural affinity of the DNA bases, such as A, G, C, and T, to couple with each other.
Moving across the sequence of letters on the DNA strands can allow scientists to make these strands of DNA to couple together in ways that produce varied shapes. It is also possible to adjust the stiffness of DNA origami which allows it to bend and coil similar to boiled spaghetti, or remain straight as a piece of dry spaghetti.
Increasing computational power and the application of DNA self-assembly for the genomics sector has considerably improved the area of DNA origami in recent years. The DNA motors could potentially be used as nanocomputers, drug delivery devices in the form of nanocapsules that open up upon reaching a site of interest, and nanorobots working on assembly lines at the nanoscale.
These applications may seem like science fiction now, but our work is helping move them closer to reality.
Alisina Bazrafshan, Study First Author and PhD Candidate, Emory University
One major difficulty of DNA motors is that the rules controlling nanoscale motion are different than those for objects perceived by humans. In fact, molecular-scale devices should be able to find their way via a steady stream of molecules. Forces like these can cause these microscopic devices to drift arbitrarily similar to pollen grains that float on a river’s surface, a phenomenon called Brownian motion.
The liquids’ viscosity also has a relatively larger effect on something as small as a molecule, and hence water turns into molasses.
Several previous DNA motors are known to “walk” with a mechanical leg-over-leg movement. But the problem is that two-legged versions are prone to be innately unstable. While walking motors with more than two legs achieve stability, the extra legs tend to slow them down.
To solve these problems, the researchers at Emory University created a rod-shaped DNA motor that rolls persistently. The motor’s “chassis” or rod contains 16 strands of DNA paired together in a four-by-four stack to create a rod that has four flat sides, similar to a wooden matchstick. From each face of the rod, 36 bits of DNA protrude just like tiny feet.
To power the motor’s movement, it is positioned on an RNA track. RNA is a nucleic acid containing base pairs that complement the DNA base pairs. After pulling at the DNA feet on one side of the motor, the RNA couples them to the track. Then, a specific RNA- targeting enzyme that is bound to DNA destroys the bound RNA rapidly. This mechanism causes the motor to roll persistently, as the DNA feet on the subsequent face of the motor get pulled in a forward direction by their affinity to RNA.
The rolling DNA motor then forges a persistent path and this makes it move in a straight line when compared to the more haphazard movement of the walking DNA motors.
Moreover, the rolling movement adds to the new speed of the DNA motor; it can move over the length of a human stem cell in less than two or three hours. DNA motors developed in the past required approximately a day to cover that same amount of distance, and a majority of them do not have the persistence to make it that far.
One major difficulty was measuring the motor’s speed at the nanometer scale. But that issue was solved by introducing fluorescent tags on both ends of the DNA motor and improving imaging conditions on a fluorescent microscope.
By trial and error methods, the team established that a rigid rod shape that offers the best solution for traveling in a straight line and that 36 feet on each motor’s face offered optimal density for speed.
“We provided a tunable platform for DNA origami motors that other researchers can use to design, test and optimize motors to further advance the field,” added Bazrafshan. “Our system allows you to test the effects of all kinds of variables, such as chassis shape and rigidity and the number and density of legs to fine tune your design.”
For example, what kinds of variables would result in a DNA motor that travels in circles? Or a motor that turns to go over barriers? Or a motor that turns in reaction to a specific target?
“We hope that other researchers will come up with other creative designs based on these findings,” concluded Bazrafshan.
The study’s co-authors include Travis Meyer, Hanquan Su, Selma Piranej, and Yuxin Duan (all are graduate students from Emory University); and Joshua Brockman and Aaron Blanchard, (graduate students in the Wallace H. Coulter Department of Biomedical Engineering, Georgia Tech and Emory).
The National Science Foundation and the National Institutes of Health funded the study.
Watch a video about the new DNA origami motor. Video Credit: Emory University.
Source: http://www.emory.edu/