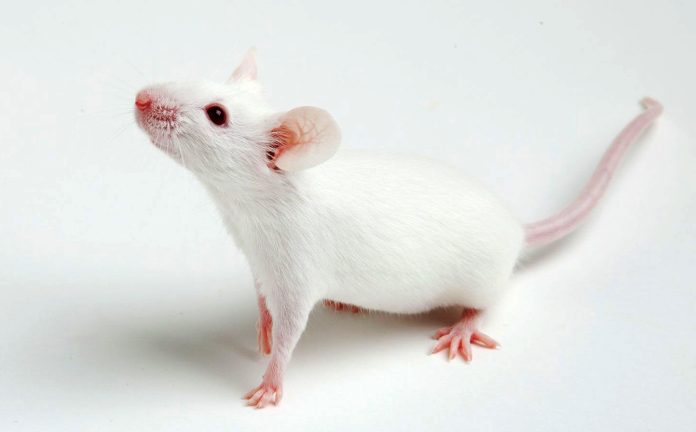
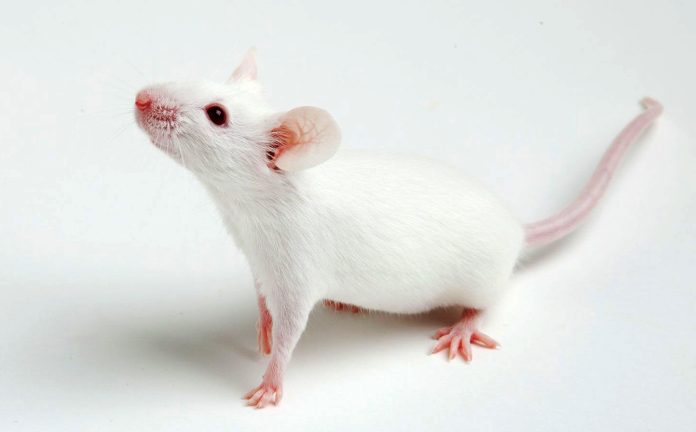
By Lieke Geerts, PhD
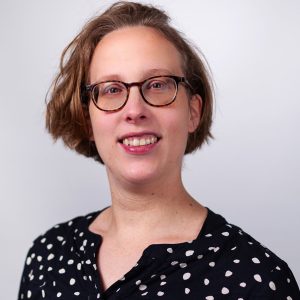
Charles River Laboratories
Ten years ago, CRISPR gene editing was introduced to the world by a paper in Science. Just eight years later, the paper’s senior authors, Jennifer A. Doudna and Emmanuelle Charpentier, shared the Nobel Prize for Chemistry. By then, it had already become abundantly clear that the gene editing tool known as CRISPR-Cas9 was transforming researchers’ ability to make precise alterations to genomes.
CRISPR has been used to manipulate the genomes of organisms in humans and animals, among other living organisms. When it comes to drug discovery and development, CRISPR is used to remove or modify DNA in research mice to study disease phenotypes and develop new treatments.
The technology streamlines the quick creation of structural variants through deletions, reproductions, or inversions of genomic regions in animal models, allowing scientists to produce more predictive cell-based models and identify new drug targets in a quicker, more cost-effective way. Sophisticated methodologies allowing for CRISPR screening to occur in the natural microenvironment—in organoid models and even in whole animal models—has exposed genetic dependencies that could not be revealed by in vitro screening efforts.
Besides the ability to create more relevant model systems relatively easily, the factor of time has also proven to be a game changer. Modeling complex human diseases requires complex genetic modifications that used to take months or years to achieve. With CRISPR, however, these modifications can be achieved in weeks. As CRISPR technology continues to evolve, it’s changing how we study animal models in major ways—transgenic mice can be generated faster than ever before and with great precision, and these animal models are instilling the ability to test and predict the efficacy and safety of a drug target early on, optimizing and accelerating the process.
1. Precision modeling that revolutionizes target validation
Animal models generated by CRISPR technology have been crucial in the discovery of new medicines. This technology generates highly customized mice and rats whose DNA has been altered to reflect a humanized immune system, which enables precision modeling in research, as well as target validation and efficacy assessment. Prior to the use of this technology, it could take up to 18 months to produce test animals—it now takes anywhere from one-third to one-half the time to create a founder animal, making it more cost-effective than alternative preclinical animal models.
CRISPR-Cas9 technology has been used to knock out DNA in research mice and rats, meaning the animal gene for a particular target is silenced and the defective gene for human disease is knocked in, introducing the same mutation into the mouse gene as we see in the human population. This allows researchers to focus on therapies for the human target.
A pre-CRISPR example of this approach can be seen in an apolipoprotein E (Apo E) knockout mouse that was manufactured by two independent laboratories in 1992. Apo E wipes away dietary and endogenous lipids and removes cholesterol from peripheral tissues. The deficiency of Apo E generates aggressive atherosclerosis, a form of cardiovascular disease, in the mouse.
The mouse with deficient Apo E showed that restricting cholesterol absorption would minimize both plasma cholesterol and the development of atherosclerosis, giving the researchers confidence to pursue clinical trials for ezetimibe, a drug later approved in 2002 for the treatment of hypercholesterolemia. Clinical trials continue to demonstrate that ezetimibe prevents major cardiovascular events in high-risk patients with preexisting cardiovascular disease.
Today, models incorporating even more precise modifications can be generated with CRISPR technology.
2. Testing efficacy in humanized systems
Predicting the efficacy of treatments is important when planning for costly and time-consuming clinical trials. Animal model systems can emulate human physiology, so these models can help generate reliable data that shows the safety and effectiveness of a treatment.
To evaluate and ensure translation between humans and animal models, CRISPR streamlines the creation of single nucleotide polymorphisms (SNPs), which are the most abundant source of genetic variation in the human genome. Accordingly, CRISPR can facilitate the use of SNPs in humanizing sizable areas of DNA in animal models and creating predictive models of disease. The ability to identify SNPs that contribute to the susceptibility of common diseases provides information that can expedite early diagnosis, prevention, and treatment of human diseases.
Researchers hoping to proceed to clinical trials for conditions such as cardiovascular disease must be able to predict the efficacy of treatments aimed at reducing morbidity and mortality. The data stemming from research with animal model systems is pivotal to efficacy predictions. Treatment efficacy can be highly impacted by genetic variation, and the introduction of disease-associated mutations in a humanized animal model can give efficacy studies a relevant background, thereby enhancing translational value even when not all genetic interactions are known.
CRISPR-Cas9-based screening can also be used to identify targets for combination therapy. For example, synthetic lethality was observed in a CRISPR screening campaign between lenvatinib treatment and the genetic inhibition of epidermal growth factor receptor (EGFR). Efficacy studies applying co-treatment of EGFR inhibitors gefitinib and lenvatinib supported these results with a good translation to advanced hepatocellular carcinoma patients who were unresponsive to first-line drug lenvatinib, showing meaningful clinical responses to the combination therapy.
3. Inspiring confidence in the safety of a given treatment
Safety is imperative in the drug development process. Before studies can move to human targets, the clinical risk profile of a potential new treatment must be predicted.
Data rendered from a reliable animal model instills trust in the technique and the safety of a particular approach. Based on results observed in these models, companies can proceed to the next stages of research and development. CRISPR-Cas9 technology can be employed at various stages for the mitigation of safety risks. Mutating and restoring a putative drug target in an isogenic environment can allow for the discrimination of off-target from on-target responses, and when this approach is applied early on, it can enable a selection of compounds with fewer off-target risks to be taken into safety studies.
Once in vivo safety studies are entered, more accurate mimicking of the complex human system helps to expose risks that may occur when moving to clinical trials, for example, by genetic variation affecting functioning of connected tissues or the immune system.
When it comes to the success of revolutionary CRISPR-based medicines, off-target effects of gene editing are pivotal. The risks of DNA deletions and unintended mutations must be considered and are an important aspect of the safety profile of such treatments. Once this is under control, a CRISPR-Cas9-driven approach can advance the use of stem cells in regenerative medicine applications such as the replacement of dysfunctional tissues or organs.
The evolving CRISPR landscape and the future of animal models
The power of the CRISPR technology lies in the unprecedented ease and control CRISPR brings to genome editing, and this power can be applied across multiple steps in the drug development pipeline. Although the technology has limitations, such as off-target effects or difficulties creating more complex genome modifications, CRISPR-Cas9 promises to advance novel medical applications, potentially treating or even curing conditions ranging from cardiovascular disease to rare inherited blood disorders.
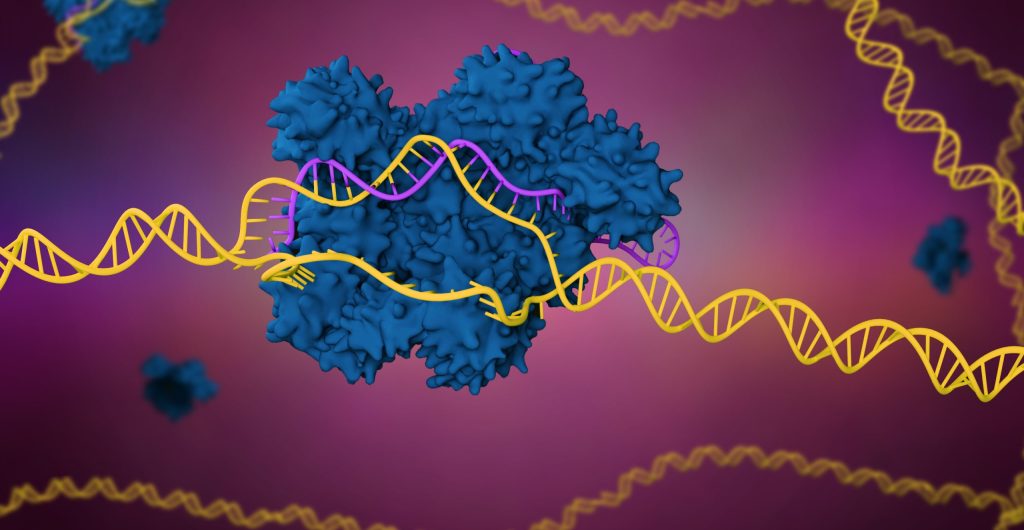
Animal models will continue to be pivotal to the research and discovery activities driving these therapies forward. However, by ensuring that in vitro models have the most relevant genetic and cellular characteristics before animal testing commences, CRISPR-Cas9 genome engineering ensures that in vivo model systems will have higher translational capacity, increasing success rates and thereby decreasing the overall use of animals.
Lieke Geerts, PhD, is a group leader at Charles River Laboratories.